The global ocean plays an important role in regulating the Earth’s climate. Throughout the oceans exist energetic rotating currents, known as ocean eddies. Their diameter ranges from 10 km near the poles to 200 km near the equator. Eddies make significant contributions to the movement of heat, salt, and carbon around the globe, which make them key processes that need to be faithfully represented in climate models.
An example of an ocean eddy is shown in the image below. This type of eddy is known as an Agulhas ring, generated at the southern tip of South Africa where the Agulhas current separates from the coast. This eddy is captured from a satellite and made visible through a phytoplankton bloom.
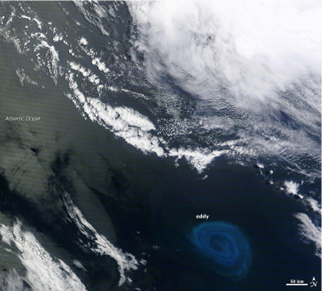
Despite the advancements in computing power, many climate models cannot directly simulate ocean eddies because their resolution is too coarse. Instead, these models use equations known as parameterisations to approximate how eddies affect the ocean circulation. One of the most common eddy parameterisations is the Gent-McWilliams (GM) formulation. This parameterisation works by mimicking the eddy transport of oceanic tracers, such as temperature and salinity.
Since the GM parameterisation was first introduced in the early 90’s, several variations have been developed, notably GEOMETRIC. The GEOMETRIC parameterisation uses an eddy energy budget to help inform the transport of tracers by ocean eddies. Studies suggest that GEOMETRIC is capable of reproducing key circulation features, particularly in the Southern Ocean. Accurately modelling the Southern Ocean is very important as it is one the largest sinks of atmospheric heat and carbon.
A parameter of the GEOMETRIC parameterisation that is difficult to constrain is the rate at which eddy energy is removed within the eddy energy budget. This term is important because an unconstrained rate of eddy energy removal can cause uncertainty in the oceans heat and carbon budgets. Eddy energy can be removed by many processes, like drag from the ocean floor, or interaction between eddies and surface winds. We focus on the interaction between eddies and wind because it is extremely important in determining a correct ocean circulation. For example, without considering this interaction, the transport of heat and mass northwards is overestimated, affecting our ability to predict long-term climate change accurately.
To address this gap in GEOMETRIC, we formulated an equation for the removal of eddy energy due to eddy-wind interaction. We made assumptions about the structure of eddies and the surface wind field to develop an equation that we could then input observational data into. The equation depends on the horizontal length of eddies; however, there is some uncertainty about what this should be in our equation. Therefore, we used two values: one that defines the scale at which rotational effects become important, and one that uses observational data from satellites. This provides an upper and lower bound for the rate of eddy energy removal.
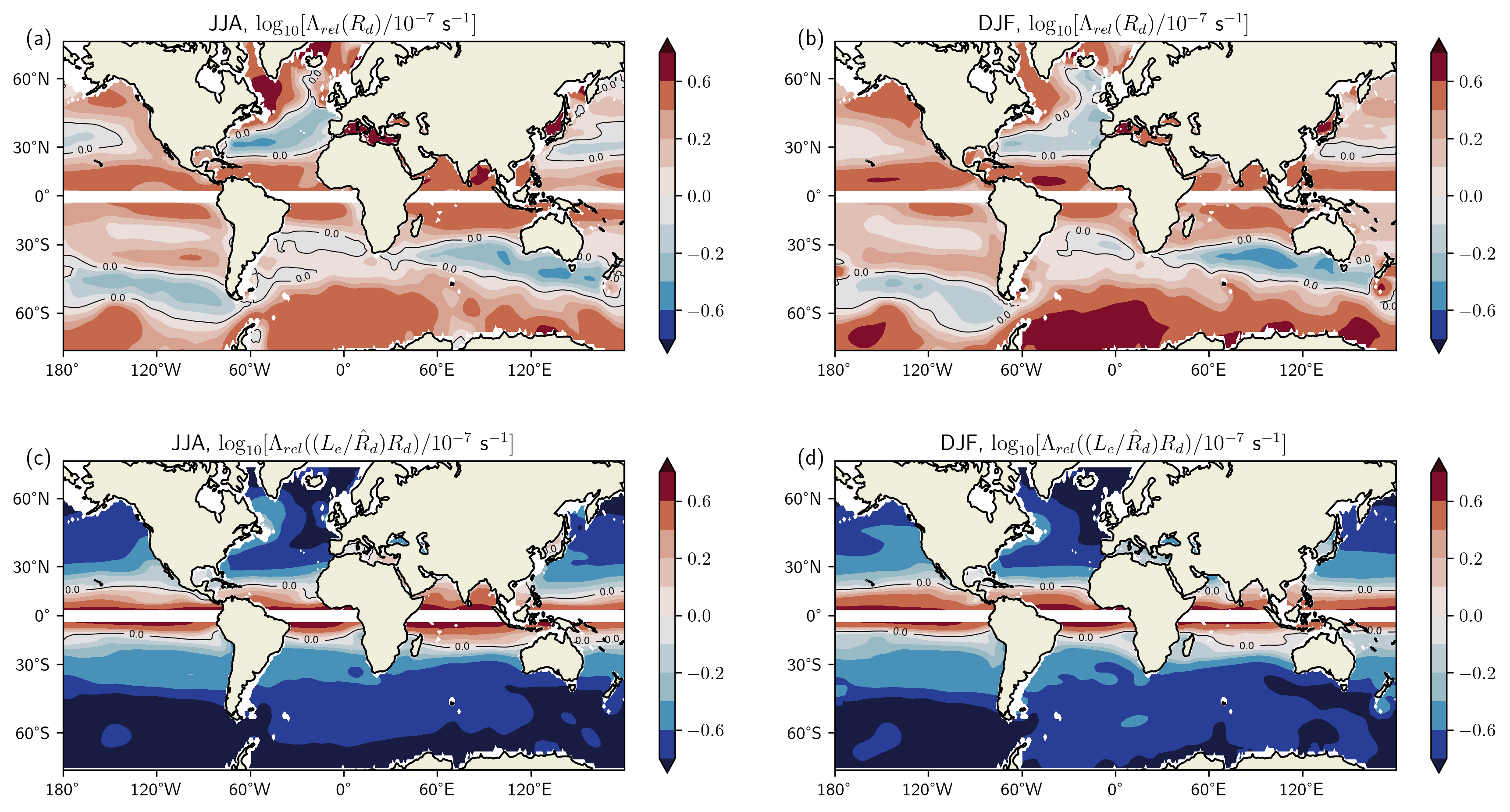
The above figure shows the rate of eddy energy removal across summer and winter seasons compared to a standard rate. The top panels show the upper bound and the lower panels the lower bound. The standard rate describes all eddy energy removal processes. Displaying this comparison on a base 10 log plot highlights where our rate is greater (red colours) or smaller (blue colours) than the standard rate. To put the values in context, and with the Southern Ocean in mind (the ocean circling Antarctica), the standard eddy energy removal rate implies it takes around 100 days to remove eddy energy. Interpreting the values in the Southern Ocean, our equation for the removal of eddy energy by surface wind varies from 0.25 (in the bottom panels) to 4 (in the upper panels) times the size of the standard rate. The larger values imply a shorter timescale for eddy energy removal.
It is interesting to speculate what these changes in eddy energy removal could mean in a simulated global climate. Changes to the timescale would alter the population of eddies and lead to changes in the global ocean circulation. In the Southern Ocean, modifying eddy mixing could have implications for air-sea exchanges of heat and carbon, supply of nutrients for life in the oceans, and the melt rate of Antarctic ice sheets.
Climate models are essential tools in our fight against a rapidly changing climate. They help us make better-informed policy choices to mitigate future climate risks. Therefore, understanding the complex interactions within the climate system and further developing state-of-the-art climate models is crucial for addressing the challenge of climate change.
Thomas Wilder is a Research Scientist at the National Centre for Atmospheric Science at the University of Reading and winner of the University’s 2024 Research Output Prize (Environment theme) for his paper as first author in Ocean Science: ‘Constraining an eddy energy dissipation rate due to relative wind stress for use in energy budget-based eddy parameterisations’.