How much the global mean temperature will change in response to increases in carbon dioxide is of high uncertainty in climate models but is critical for future planning and climate adaptation. Jian-Feng Gu describes a new understanding of how the vertical motions of heat and moisture within shallow cumulus clouds can be affected by pressure change due to small-scale thermals. This can help improve the representation of ‘vertical transport’ in cumulus clouds in climate models and potentially help explain the differences between climate models in how they predict warming.
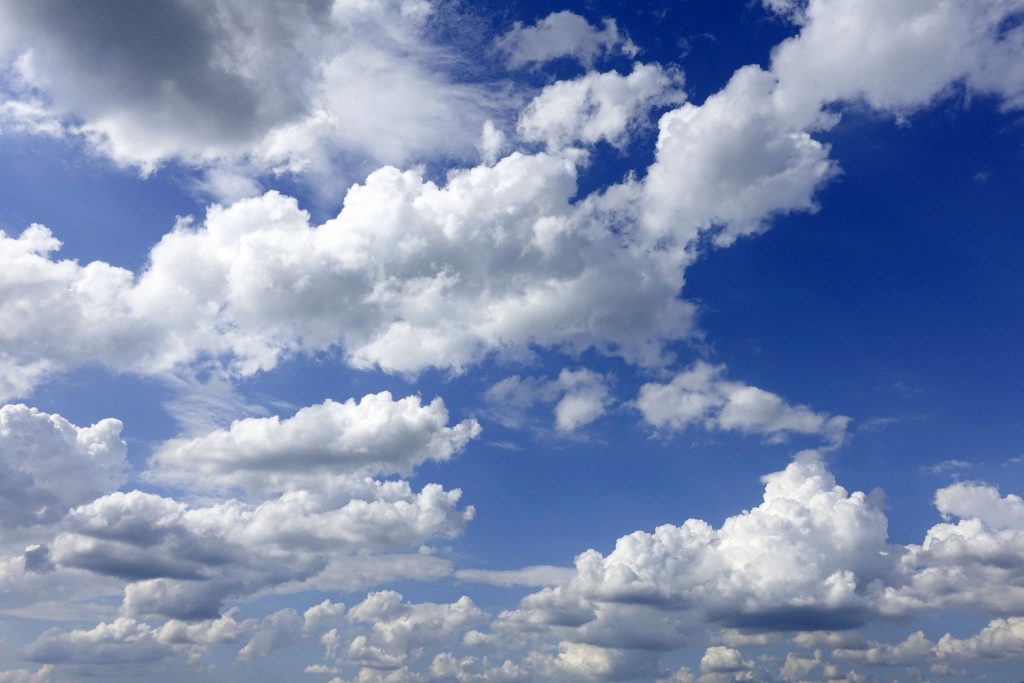
In this study, we looked at shallow cumulus clouds – the low-level fluffy cotton-like clouds – that generally cool the earth by reflecting the incoming solar radiation and bringing moisture to the low-level atmosphere by vertically transporting heat and water vapour. We created a very high-resolution numerical simulation to explore how the vertical motion within clouds is affected by small-scale thermals. This knowledge is crucial for a reasonable representation of vertical mixing by shallow clouds in climate models.
The vertical transport of heat and moisture associated with shallow cumulus clouds is one of the most important factors that can help explain the differences between climate models in how they predict warming response to the doubling of carbon dioxide in the earth’s atmosphere. Cumulus clouds are formed through a sequence of rising warm thermals, but they are generally too small to be explicitly resolved in current climate models, which usually have horizontal resolutions of 10 to 100km.
The solution is to build a simplified model (a parameterization scheme) to describe the contribution of vertical transport from a large number of clouds. Most parameterizations of the vertical velocity within shallow cumulus clouds are based on a steady state, conditionally averaged vertical momentum equation, which is designed to describe the collective behaviour of an ensemble of clouds. In the vertical momentum equation, buoyancy (the density difference between the clouds and surrounding environment) is the main driver of vertical motion and is balanced by the pressure gradient force (air pressure difference in the vertical) whose importance has recently been recognised. Current understanding of the deceleration effect for updraft has mainly focused at the scale of single cloud or the even finer scale of thermals. Little is known about how the pressure effect on thermals can be related to that on a large ensemble of clouds.
In our study, we performed a large eddy simulation of shallow cumulus clouds using the Met Office-NERC Cloud Model based on the Barbados Oceanographic and Meteorological Experiment. The resolution of this simulation is high enough to resolve the most energetic structures that are responsible for vertical transport associated with shallow cumulus clouds. We developed an algorithm to track the life cycle of each shallow cloud in the simulation, and reviewed the steady state vertical momentum of the cloud ensemble and individual clouds to establish the relationship between them.
We found that the pressure drag for a cloud ensemble is primarily controlled by the air mass redistribution through flows (the dynamical component), rather than the air pressure difference in response to temperature difference (the thermodynamic component. The dominance of dynamical pressure drag and its increased magnitude with height are common features of individual clouds except that the total drag of a single cloud over life cycle presents vertical oscillations (frequency small and large tendencies in vertical, or positive-negative couplets in vertical). These oscillations are usually associated with rising thermals but complicated by the evaporation-driven downdrafts outside the cloud. The horizontal vorticity is amplified as the thermals rise to higher altitudes due to continuous baroclinic (horizontal buoyancy gradient) vorticity generation. This leads to the increased magnitude of local minima of dynamical pressure perturbation with height and consequently to increased dynamical pressure drag.
This study is the first to bridge the gap between our understanding of the pressure drag of individual thermals and the ensemble of clouds. It shows that deceleration effect can be well understood by combining the dynamics of individual thermals: that is, a self-induced increasing resistance of upward motion with height through the redistribution of air mass. This opens the door to much better treatments of the ‘deceleration’ effect for shallow cumulus clouds in climate models and thus hopefully help understand the climate sensitivity and plan for climate change.
Jian-Feng Gu is a Post-doctoral Research Assistant in Meteorology and runner-up for the University’s Early Career Researcher Output Prize for his article, ‘Pressure drag for shallow cumulus clouds: From thermals to the cloud ensemble’ in Geophysical Research Letters, 47 (2020).